Mendel's Experiments and Heredity: Inheritance Patterns and Genetic Crosses in Pea Plants
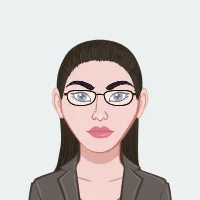
Mendelian genetics, named after Gregor Mendel, the father of modern genetics, forms a foundational pillar in understanding how traits are passed from one generation to the next. This guide aims to help university students navigate through genetic assignment questions on Mendel's experiments and the principles of heredity, focusing on practical examples and explanations.
Understanding Mendel's Hybridizations
Mendel's work on hybridizations involved careful manipulation of plant breeding. He transferred pollen from the anther of a male plant to the stigma of a female plant. This meticulous process allowed him to control which plants were cross-pollinated, leading to significant discoveries in inheritance patterns. For example, Mendel observed traits like seed texture, one of the seven characteristics he studied in pea plants. His choice of pea plants, with their easily observable traits and controlled pollination process, provided clear and replicable results that were critical in formulating the laws of inheritance.
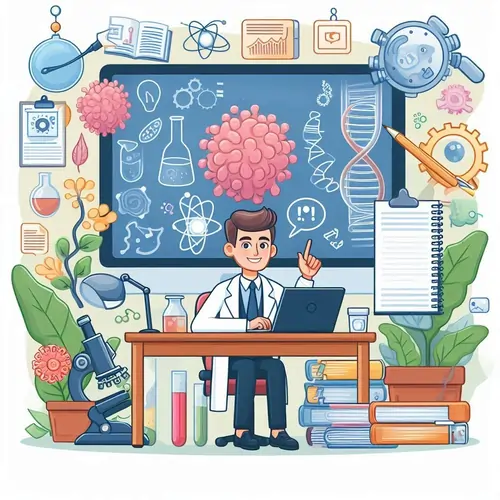
Predicting Offspring Phenotypes and Genotypes
A crucial part of understanding genetics is predicting the phenotypes (observable traits) and genotypes (genetic makeup) of offspring. For instance, when true-breeding pea plants with green seeds are crossed with those having yellow seeds, and given that yellow is the dominant trait, all F1 offspring will exhibit yellow seeds. This occurs because the dominant allele (Y) masks the expression of the recessive allele (y) in the heterozygous genotype (Yy).
Further complexity arises in predicting the results of dihybrid crosses, such as those involving pod texture (inflated vs. constricted). If we assume a 3:1 dominant-to-recessive phenotypic ratio, which Mendel consistently found, then in a generation where 650 plants have inflated pods, approximately 217 plants are expected to have constricted pods. This ratio illustrates Mendel's law of segregation, where alleles for a trait separate during gamete formation.
Exploring Phenotypes and Genotypes
The distinction between phenotype and genotype is fundamental in genetics. The phenotype refers to the observable characteristics, such as flower color or seed texture, while the genotype describes the organism's specific genetic makeup. A recessive trait will only manifest phenotypically when an individual is homozygous for that allele, meaning both alleles are identical and recessive (e.g., rr for a trait controlled by the 'r' allele).
Moreover, different inheritance patterns, such as incomplete dominance, can lead to a blend of traits in the offspring. An example is seen in the mating of true-breeding black and white mice, resulting in all gray offspring. This gray coloration indicates incomplete dominance, where neither allele is fully dominant, and the heterozygous phenotype is an intermediate blend.
Inheritance Patterns
Inheritance patterns become even more intricate with phenomena like codominance and multiple alleles, as demonstrated in human ABO blood groups. In this system, the IA and IB alleles are codominant, meaning both are expressed equally in individuals with type AB blood. This pattern is distinct from simple dominance and provides a classic example of how multiple alleles can combine to determine phenotype.
In studying these patterns, it’s important to consider the expected outcomes in genetic crosses. For example, in a cross involving heterozygous parents for a recessive lethal allele, the ratio of genotypes observed among surviving offspring would be 1 homozygous dominant to 2 heterozygous. The homozygous recessive genotype is typically not observed due to lethality before birth.
Dihybrid and Trihybrid Crosses
Dihybrid and trihybrid crosses, which involve two or three traits respectively, are used to study the inheritance of multiple traits simultaneously. For instance, in a dihybrid cross between individuals heterozygous for two traits (AaBb), the expected ratio of F1 gametes (AB, Ab, aB, ab) is 1:1:1:1. This ratio stems from Mendel's law of independent assortment, which states that genes for different traits segregate independently during gamete formation.
The complexity increases with trihybrid crosses, which involve three traits and yield 64 possible genotypes and 16 phenotypes due to combinations of dominant and recessive alleles. Understanding these patterns helps in predicting the distribution of traits in offspring and demonstrates the power of Mendel's laws in explaining genetic inheritance.
Practical Applications
The practical application of Mendelian genetics extends beyond theoretical problems to real-world examples. For instance, using a Punnett square to predict the offspring of a cross between a homozygous recessive dwarf pea plant and a heterozygous tall pea plant can reveal a 1:1 phenotypic ratio of tall to dwarf plants. Such exercises reinforce the concepts of dominant and recessive traits and the probability of different outcomes.
Epistasis, another important genetic principle, involves one gene masking or modifying the expression of another gene. This concept can be illustrated with examples such as the summer squash, where a cross between white heterozygotes (WwYy) results in a phenotypic ratio of 12 white:3 yellow:1 green. This result is due to one gene influencing or suppressing the expression of another, a phenomenon critical in understanding complex traits.
Conclusion
Navigating Mendelian genetics requires a solid understanding of the foundational principles that Gregor Mendel established through his pioneering experiments. By mastering concepts such as dominant and recessive traits, inheritance patterns, and the use of Punnett squares for predicting genetic outcomes, students can effectively tackle a wide range of biology assignment questions.
The ability to analyze genetic crosses, predict phenotypic ratios, and understand complex inheritance patterns, such as incomplete dominance and codominance, equips students with essential skills for studying genetics. Furthermore, grasping the principles of epistasis and dihybrid or trihybrid crosses enhances their capacity to tackle more advanced genetic problems.
Overall, a thorough comprehension of Mendelian principles not only facilitates success in academic assignments but also fosters a deeper appreciation of genetic mechanisms that govern biological inheritance. By applying these principles to practical problems and exercises, students can achieve a clearer and more integrated understanding of genetics, paving the way for further exploration and discovery in the field.