Understanding Microevolution, Macroevolution, and Population Genetics
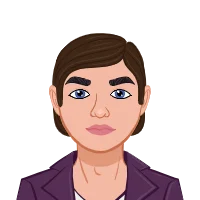
Understanding the intricate details of evolutionary biology can be challenging, especially when it comes to solving biology assignment that test your grasp of key concepts. This guide will help university students tackle common questions related to microevolution, macroevolution, population genetics, and more. By breaking down each topic and providing thorough explanations, you'll be better equipped to understand and answer these questions in your assignments.
Microevolution vs. Macroevolution
A foundational concept in evolutionary biology is the distinction between microevolution and macroevolution. Microevolution refers to small-scale changes within a population over a short period, involving variations in allele frequencies due to mechanisms like natural selection, genetic drift, mutation, and gene flow. On the other hand, macroevolution encompasses larger-scale evolutionary changes that result in the formation of new species and taxa over long periods. This involves the accumulation of microevolutionary changes that lead to significant differences, such as speciation events. Understanding this distinction is crucial for explaining how small genetic changes can eventually lead to the emergence of new species.
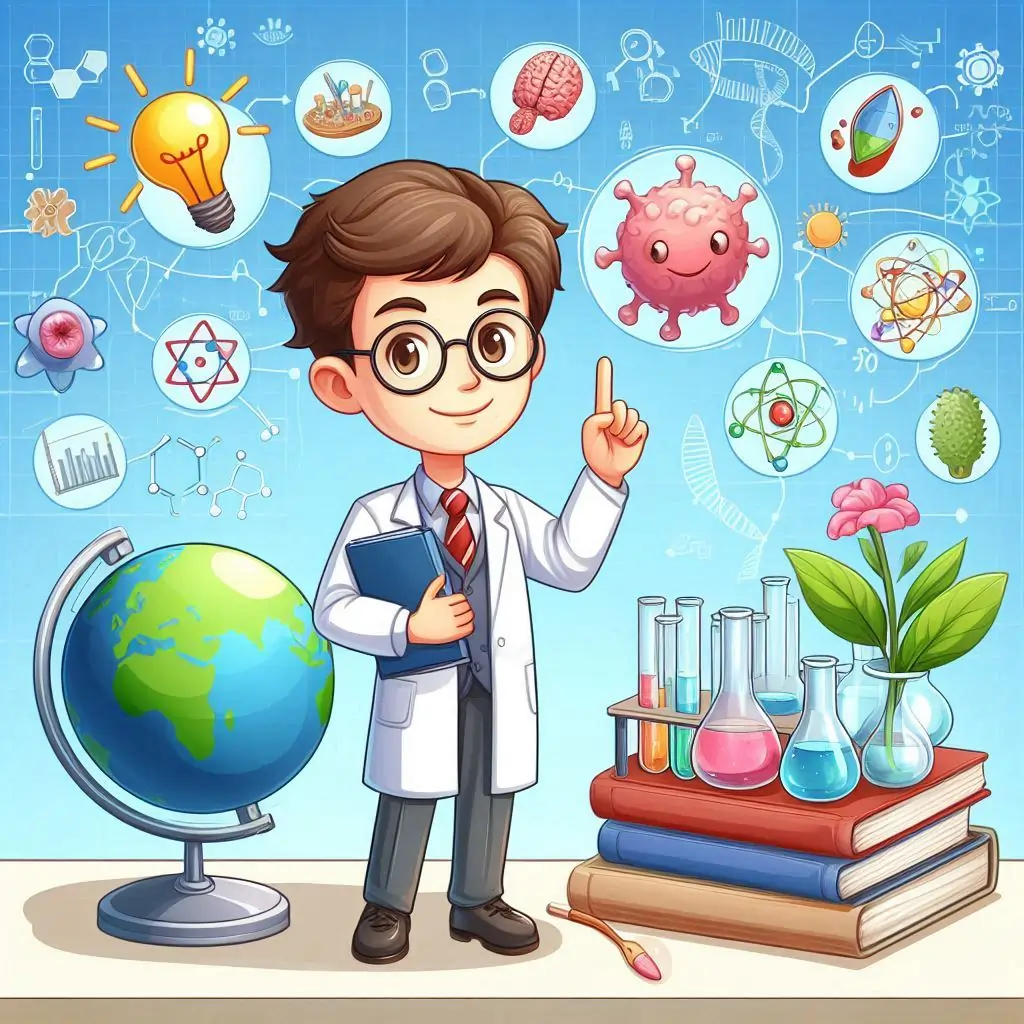
The Role of Population Genetics
Population genetics is the study of how selective forces change the allele frequencies in a population over time. It focuses on understanding how the genetic composition of a population changes due to factors like natural selection, genetic drift, mutation, and gene flow. This field provides the mathematical framework to describe and predict the genetic structure of populations, helping biologists understand the underlying genetic diversity and evolutionary potential of populations.
Hardy-Weinberg Equilibrium
A key principle in population genetics is the Hardy-Weinberg equilibrium, which states that allele and genotype frequencies in a population remain constant from generation to generation in the absence of evolutionary influences. For a population to be in Hardy-Weinberg equilibrium, it must meet five conditions: no mutations, random mating, no gene flow, infinite population size, and no selection. This principle serves as a baseline to determine if a population is evolving. Any deviations from the equilibrium conditions suggest that one or more evolutionary forces are at work.
Founder Effect and Genetic Drift
The founder effect and genetic drift are important concepts explaining how allele frequencies can change due to random sampling effects. The founder effect occurs when a new population is established by a small number of individuals, carrying only a fraction of the genetic variation from the original population. This can lead to significant differences in allele frequencies compared to the original population. Genetic drift refers to random changes in allele frequencies, which are more pronounced in small populations. Both mechanisms can lead to significant evolutionary changes over time, especially in isolated populations.
Gene Flow in Populations
Gene flow, or the transfer of alleles from one population to another, plays a crucial role in maintaining genetic diversity within populations. It occurs when individuals from different populations interbreed, introducing new alleles into the gene pool. This exchange of genetic material can alter allele frequencies, enhance genetic variation, and reduce differences between populations, promoting adaptation and evolutionary potential.
Introducing Genetic Variation
Mutations and gene flow are two primary evolutionary forces that introduce new genetic variation into a population. Mutations are changes in DNA that introduce new alleles, providing the raw material for evolution. Gene flow brings new alleles from other populations, increasing genetic diversity. Both processes are essential for generating the genetic variation necessary for populations to adapt to changing environments.
Assortative Mating
Assortative mating occurs when individuals preferentially mate with others that are similar to themselves in certain traits. This non-random mating pattern can affect genotype frequencies within a population, leading to an increase in genetic similarities among mates. Assortative mating can accelerate the evolution of specific traits and contribute to reproductive isolation and speciation.
The Impact of Inbreeding
Inbreeding, or mating between closely related individuals, can have significant genetic consequences. It increases the probability that offspring will inherit deleterious recessive alleles from both parents, leading to reduced fitness. This phenomenon, known as inbreeding depression, results from the increased expression of harmful recessive traits. Understanding the impact of inbreeding is crucial for managing the genetic health of small or isolated populations.
Understanding Clines
A cline is a gradient in a trait of a species across a geographic area, often correlated with an environmental gradient. Clines illustrate how populations adapt to varying environmental conditions over a geographic range. For example, body size in mammals often increases with latitude, following Bergmann's rule. Studying clines helps biologists understand how environmental factors drive genetic and phenotypic variation within species.
Types of Selection
Different types of selection can shape genetic variation within populations. Diversifying selection, for instance, favors extreme values for a trait over intermediate values, increasing genetic variance. This type of selection can lead to the emergence of distinct phenotypes within a population. In contrast, stabilizing selection favors intermediate traits, reducing genetic variance, while directional selection favors one extreme phenotype, shifting the population's genetic makeup in a particular direction.
Sexual Dimorphism
Sexual dimorphism refers to differences in appearance or behavior between males and females of the same species. These differences often arise from sexual selection, where traits that enhance reproductive success are favored. Understanding sexual dimorphism provides insights into the evolutionary pressures that shape mating behaviors and reproductive strategies.
Good Genes Hypothesis
The good genes hypothesis explains why individuals with advantageous traits are more likely to attract mates and reproduce. According to this hypothesis, traits that indicate good health and genetic quality are preferred by mates, leading to the selection of beneficial alleles. This process enhances the overall fitness of the population by promoting the proliferation of advantageous traits.
Solving Critical Thinking Questions
To effectively solve critical thinking questions in evolutionary biology, it's essential to apply the concepts discussed above. For example, calculating the genetic structure of a population involves determining allele frequencies and applying the Hardy-Weinberg principle. Understanding the bottleneck effect requires recognizing how population size reductions impact genetic diversity. Describing natural selection involves identifying real-world examples of traits that confer a survival advantage. By mastering these concepts, students can confidently tackle a wide range of evolutionary biology questions and deepen their understanding of the subject.
Conclusion
Grasping the nuances of evolutionary biology assignment is crucial for students. By breaking down complex concepts such as microevolution and macroevolution, population genetics, the Hardy-Weinberg principle, genetic drift, gene flow, and various types of selection, this guide has provided a comprehensive framework to approach and solve typical assignment questions.
Understanding these foundational principles not only aids in answering specific questions but also enhances overall comprehension of evolutionary processes. This, in turn, enables students to analyze real-world biological phenomena more effectively. By applying the explanations and examples provided here, students can confidently tackle their assignments, deepening their knowledge and preparing them for more advanced studies in evolutionary biology. Remember, mastering these concepts takes practice and engagement with the material, so continue to explore and question as you progress in your studies.